Anatomy of CNS
Anatomical Regions of the Brain

The nervous system is the network of interlinked nerve cells that coordinates all the sensory and motor activities of the body. It is responsible for controlling our speech and behavior and for regulating the activity of all internal organs and body systems. Anatomically, the nervous system is often considered as two parts: the central nervous system—CNS—and the peripheral nervous system. The brain is part of the CNS. Select the image of the brain to learn more.
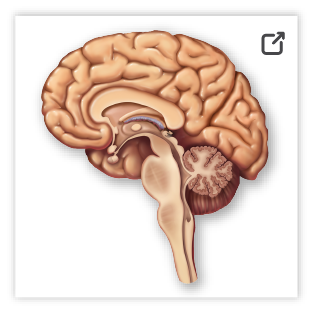
Lobes of the Brain

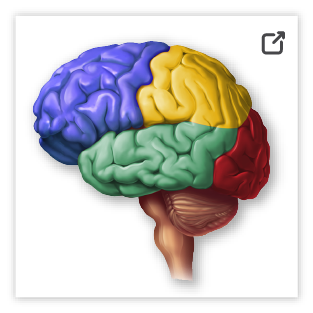
Each cerebral hemisphere is divided into lobes. Although some sources list two additional—small—lobes, the four that are most important are shown on this screen. Select the image of the brain to learn more.
The Cerebrum

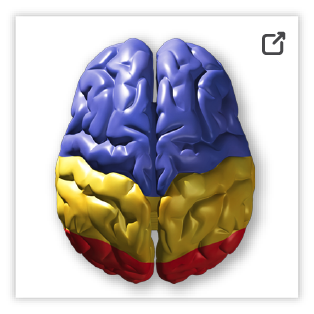
Although the two hemispheres seem to be mirror images of each other, they are different. For instance, the ability to form words seems to lie primarily in the left hemisphere, while the right hemisphere seems to control many abstract reasoning skills. Nearly all of the signals from the brain to the body, and vice versa, cross over on their way to and from the brain. Select the brain to show a magnified view of the cerebrum.
Internal Structures of the Brain

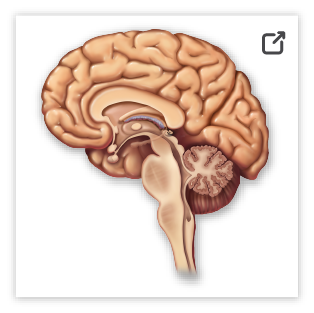
If we go down just a little deeper into the brain, we could see four different structures that work with the cerebrum to accomplish both conscious and subconscious tasks during the day and night. One of these, the corpus callosum, has an interesting job of connecting the left side of the brain with the right side. Can you imagine what it would be like if the two hemispheres were not connected? A lot of things would be nearly impossible to do. Select the image of the brain to learn more about each component.
Basal Ganglia

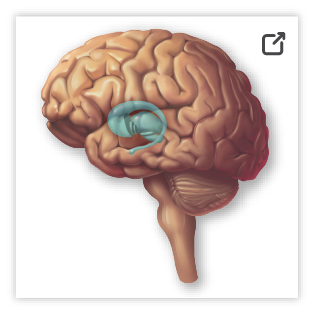
The basal ganglia consist of several nuclei of gray matter found buried within the white matter of the cerebral hemispheres. There are four principal nuclei: subthalamic nucleus, substantia nigra, striatum, and globus pallidus. The basal ganglia help initiate and terminate movements of the body. They also suppress unwanted movements and regulate muscle tone. In addition, the basal ganglia influence many aspects of cortical function, including sensory, limbic, cognitive, and linguistic functions. Select the brain to show a magnified view of the basal ganglia.
The Limbic System

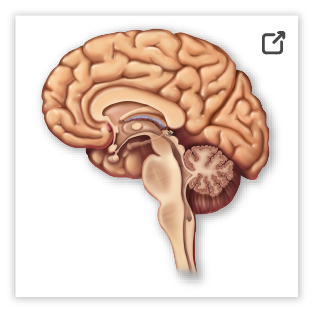
The limbic system is a functional network rather than an anatomical structure. Nerve fibers are contributed to it from several parts of the brain. The circuitry of the limbic system includes parts of the prefrontal cortex, basal ganglia, the thalamus, and a structure called the amygdala. This set of structures, together with the hippocampus and cingulate cortex, is generally referred to as the limbic system. Select the brain to show a magnified view of the limbic system.
Microstructures of the Brain

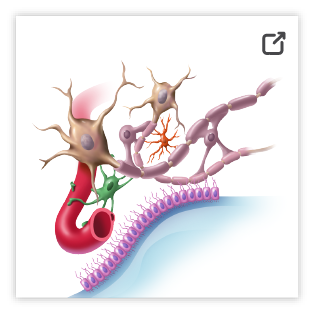
The cerebral cortex contains neurons and supporting cells called neuroglial cells. There are several different types of neurons, and we will focus on these in the next screen. Neuroglial cells—sometimes just called glial cells—are non-neuronal cells in the brain, and they perform a variety of functions. The different types of glial cells include oligodendrocytes, microglia, and astrocytes. Select the image to show a magnified view of microstructures of the brain.
Neurons

A neuron is a nerve cell that is the basic building block of the nervous system. Neurons are similar to other cells in the human body in a number of ways, but there is one key difference between neurons and other cells: neurons are specialized to transmit information throughout the body.
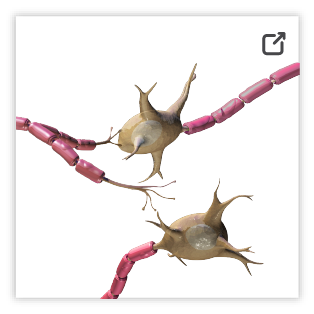
These highly specialized nerve cells are responsible for communicating information as both chemical and electrical stimuli. There are also several different types of neurons responsible for different tasks in the human body. Sensory neurons carry information from the sensory receptor cells throughout the body to the brain. Motor neurons transmit information from the brain to the muscles of the body. Interneurons are responsible for communicating information between different neurons in the body. Select the neuron image to show a magnified view.
Progress Check Questions
What Is a Neurotransmitter?
A neurotransmitter is a chemical messenger that carries, boosts, and modulates signals between neurons and other cells in the body. Neurotransmitters released from synaptic vesicles located in presynaptic axon terminals act on a large variety of receptor molecules to elicit fast excitation or inhibition as well as slower modulatory effects on neuronal excitability and responsiveness to other neurotransmitters. In addition to electrophysiologic effects, chemical transmission triggers long-term effects on function, protein expression, and structure of the target cell. These effects are critical for dynamic, use-dependent, long-term changes in the efficacy of synaptic transmission.

Three Neurotransmitters
The Synapse
There are two major types of neurotransmission: (1) chemical neurotransmission; and (2) electrochemical neurotransmission. In chemical neurotransmission, the first neuron secretes at its nerve-ending synapse a chemical substance called a neurotransmitter (or often called simply a transmitter substance). The neurotransmitter binds to the receptor proteins in the membrane, either activating, inhibiting, or modulating the activity of the receptors.

The synapse is like a gap or space between the sender and the receiver in the nervous system. If all of the nerves were physically connected, it is possible that the messages to and from the brain would be sent too fast for a proper response. What the synapse does is provide a little buffer space so that the neurotransmitter (the messenger) has to take the time to travel across before the receiver gets the message. Now this isn’t very long in reality, but it allows for some pacing of messages that works better for timing purposes. Select neuron to learn more.
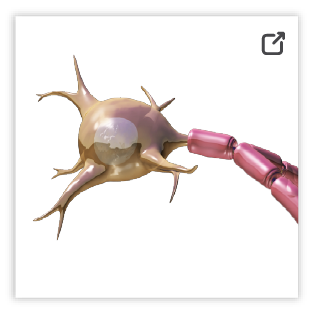
Chemical Transmission
Chemical neurotransmission is a process by which a presynaptic cell, on excitation, releases a specific chemical agent—a neurotransmitter—to cross a synapse to activate, inhibit, or modify receptors in the postsynaptic cell.
Select any of the four labels to learn about the role of each in chemical transmission.
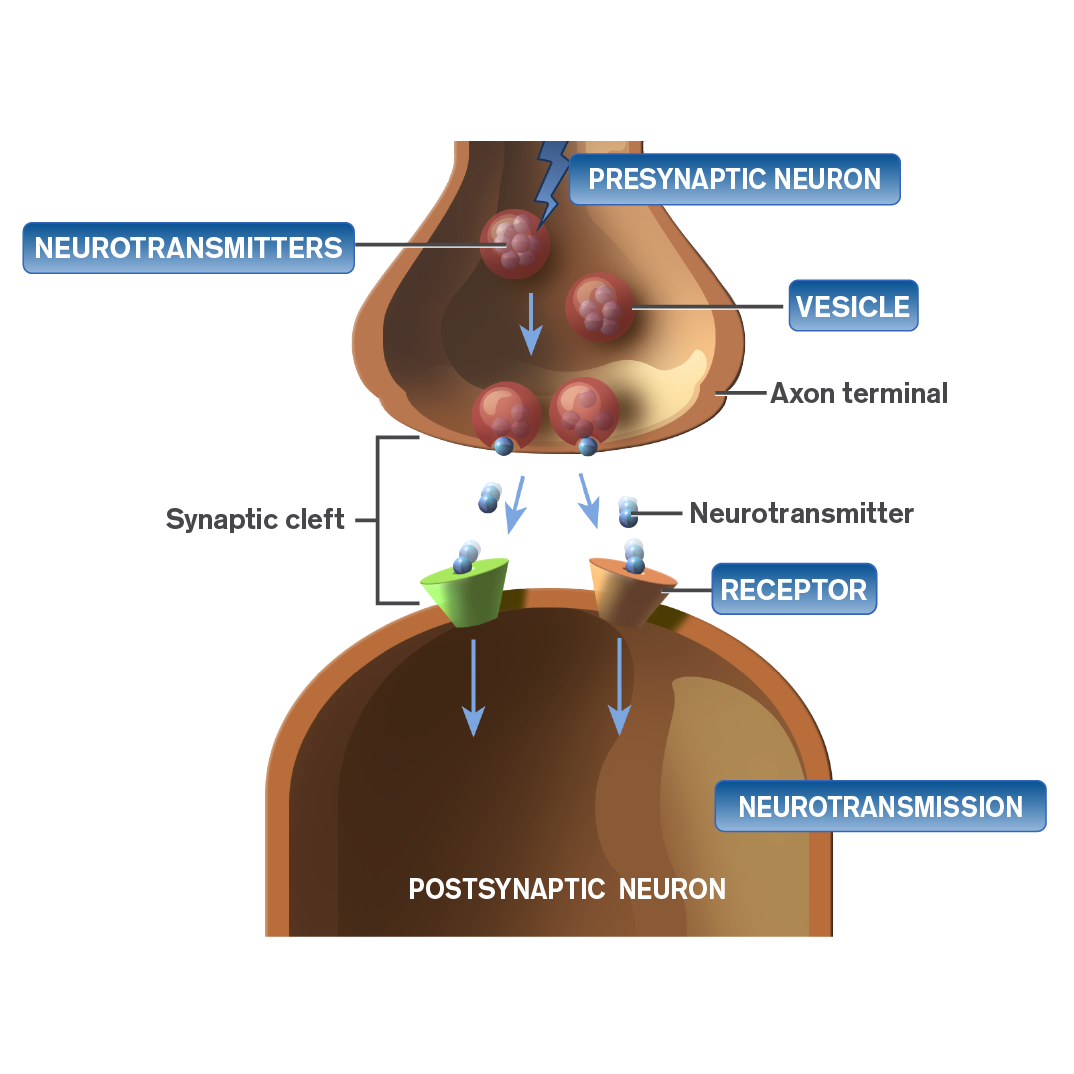
Neurotransmitters
The neurotransmitters cross the synaptic cleft and bind to specific receptors on the postsynaptic neuron.
There are two main classes of chemical substances that serve as neurotransmitters and neuromodulators: small-molecule transmitters and large-molecule transmitters. Small-molecule transmitters include amino acids, such as glutamate, GABA, and glycine; acetylcholine; monoamines, such as norepinephrine, epinephrine, dopamine, and serotonin; and adenosine triphosphate or ATP. Large molecule transmitters include a group of substances called neuropeptides, which will not be covered in this module.
Presynaptic neuron
A potential electrical signal reaches the axon terminal of the presynaptic neuron.
Vesicle
A vesicle is a membrane-enclosed sac that stores or transports substances. Vesicles fuse with the cell membrane of the presynaptic neuron to release stored neurotransmitters into the synaptic cleft.
Receptor
This is a protein molecule within a cell membrane that has specific binding sites for select neurotransmitters or drugs and which produces a specific physiological effect.
Dopamine and Dopamine Receptors

Dopamine is an important neurotransmitter involved in the pathophysiology of schizophrenia and major depressive disorder (MDD) and is involved in four different neural pathways: mesocortical, mesolimbic, nigrostriatal, and tuberoinfundibular.
There are two major types of dopamine receptors. The neurotransmitter receptor proteins are integral components in the communication between adjacent cells of the nervous system, spanning the width of the postsynaptic membrane and protruding into the synaptic cleft and cell cytoplasm. They mediate the effects of neurotransmitters between adjacent neurons.
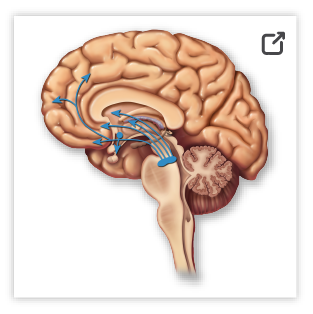
Although it was initially believed that each neurotransmitter interacted with a single type of receptor, it is now clear that there exists a family of neurotransmitter receptors for each neurotransmitter, called subtypes. Thus, depending on which receptor subtype the neurotransmitter binds with on the postsynaptic neuron, dopamine can increase or decrease cellular activity. Select the image of the brain to learn more about the four dopaminergic pathways.
Serotonin and Serotonin Receptors
Serotonin—also known as 5-HT—is a neurotransmitter that is found throughout the body.
There are multiple receptor subtypes for serotonin; the roles of these receptor subtypes are not fully elucidated. Serotonergic neurons project widely throughout the brain from their origin in the brain stem.
The wide dispersal of serotonergic neurons throughout the brain enables serotonin to be involved in modulating diverse body functions.
High concentrations of serotonin are found in the CNS, platelets, and certain cells in the gastrointestinal tract.
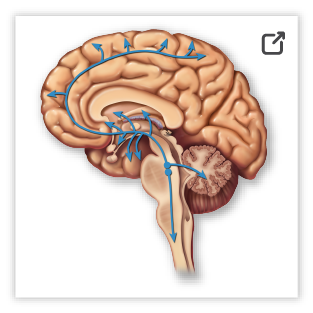
The raphe nuclei are the group of nuclei in the brain stem that releases serotonin into the brain. Select the brain to learn more about serontonin pathways and body functions.
Norepinephrine and Norepinephrine Receptors

Norepinephrine Pathways
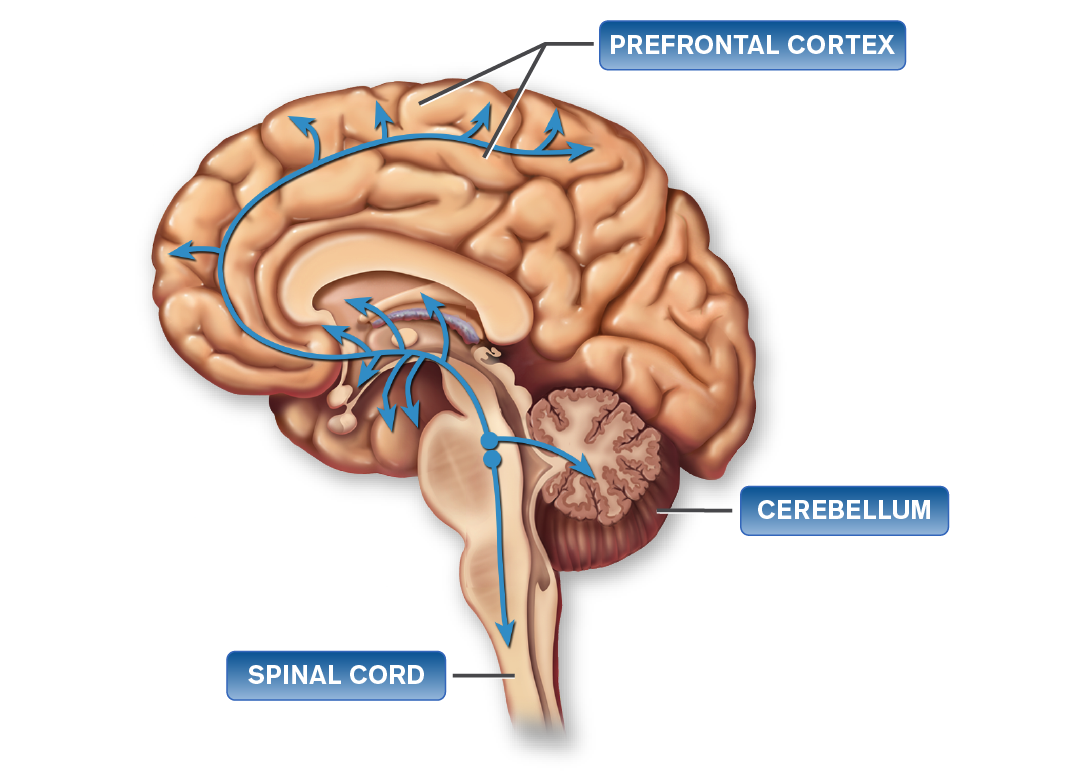
Prefrontal cortex
Some noradrenergic projections to the prefrontal cortex are thought to help regulate mood; others are thought to mediate attention.
The noradrenergic projection to the limbic cortex is thought to mediate emotions, energy, fatigue, and psychomotor agitation/retardation.
Cerebellum
The noradrenergic projection to the cerebellum is thought to mediate motor movements, especially tremor.
Spinal cord
The noradrenergic projection to the brain stem controls blood pressure.
Select each of the three norepinephrine pathway labels to learn more.
Receptor Subtypes
Dopamine | Dopaminergic receptors (D1-5 subtypes) |
Serotonin | 5-HT receptors (5-HT1A-F, 5-HT2A-C, 5-HT3-7 subtypes) |
Noradrenaline | α-adrenergic receptors (α1A-C, α2A-C subtypes) |
Glutamate | β-adrenergic receptors (β1-3 subtypes) |
GABA | Ionotropic receptors: non-NMDA (AMPA, kainate), NMDA receptors |
Neurotransmitters are released and diffuse across the synapse and bind to structures called receptors located within the cell membrane of the dendrite of the adjacent nerve cell. The table shown on this screen shows the types of receptors—and receptor subtypes—to which the major neurotransmitters bind. Note that for each neurotransmitter there are several important receptor subtypes. Keep this in mind as you learn about how an imbalance in neurotransmitters plays a role in MDD and schizophrenia and the rationale for the design of various drugs to treat these disorders.

Drug-Receptor Interaction

Agonism, Antagonism, and Partial Agonism
Progress Check Questions
References
- Purves D, Augustine G, Fitzpatrick D, Hall W, LaMantia A, White L. Neuroscience. Sunderland, MA: Sinauer Associates, Inc.; 2004.
- Tortora G, Derrickson B. Principles of Anatomy & Physiology. Hoboken, NJ: John Wiley & Sons, Inc.; 2009.
- Stedman's Online (Medical Dictionary). 2017. http://www.stedmansonline.com. Accessed September 9, 2017.
- Oberheim N, Goldman S, Nedergaard M. Heterogeneity of astrocytic form and function. Methods Mol Biol. 2012:814:23-45.
- Benarroch E. Chapter 8: Neurotransmitters. In: Waldman S, Terzic A, eds. Pharmacology and Therapeutics: Principles To Practice. 1st ed. Philadelphia, PA: Elsevier; 2009.
- 11US15IBS0060 Brex Training Program: HS103 Neuroanatomy Module April 2015.
- Chapter 10: Cerebral Hemispheres/Telencephalon. In:Waxman SG, ed. Clinical Neuroanatomy. 27th ed. New York, NY: McGraw-Hill; 2013.
- Chapter 9. Diencephalon. In: Waxman SG, ed. Clinical Neuroanatomy. 27th ed. New York, NY: McGraw-Hill; 2013.
- Chapter 7. The Brain Stem and Cerebellum. In: Waxman SG, ed. Clinical Neuroanatomy. 27th ed. New York, NY: McGraw-Hill; 2013.
- Crossman AR, Neary D. Chapter 20. Cerebellum. In: Standring S, ed. Gray's Anatomy. 40th ed. London: Elsevier Health Sciences UK; 2008.
- Crossman AR, Neary D. Chapter 18. Spinal Cord: internal organization. In: Standring S, ed. Gray's Anatomy. 40th ed. London: Elsevier Health Sciences UK; 2008.
- Martin J. Chapter 1. Introduction to the central nervous system. In: Martin J, ed. Neuroanatomy. 3rd ed. New York, NY: McGraw-Hill; 2003.
- Brain Basics. National Institute of Neurological Disorders and Stroke. April 2001. https://catalog.ninds.nih.gov/pubstatic//01-3440A/01-3440A.pdf. Accessed September 9, 2017.
- Hartwig M, Wilson L. Chapter 50. Anatomy and physiology of the nervous system. Price S, Wilson L, eds. Pathophysiology: Clinical Concepts of Disease Processes. 6th ed. St. Louis, MO: Mosby; 2003.
- R. Chapter 9. Limbic System. In: Swenson R, ed. The Review of Clinical and Functional Neuroscience. 2006. http://www.dartmouth.edu/~rswenson/NeuroSci/chapter_9.html. Accessed September 9, 2017.
- Guyton A, Hall J. Behavioral and motivational mechanisms of the brain—the limbic system and the hypothalamus. In: Guyton A, Hall J, eds. Textbook Of Medical Physiology. 11th ed. Philadelphia, PA: Elsevier Saunders; 2007:Chapter 58.
- Purves D, Williams S. The limbic system. In: Purves D, Augustine GJ, Fitzpatrick D, et al, eds. Neuroscience. 2nd ed. Sunderland MA: Sinauer Associates; 2001. https://www.ncbi.nlm.nih.gov/books/NBK10799/. Accessed September 12, 2017.
- Stufflebeam R. Neurons, synapses, action potentials, and neurotransmission. The Mind Project [Web site]. http://www.mind.ilstu.edu/curriculum/neurons_intro/neurons_intro.php. Accessed September 10, 2017.
- Hall J. Chapter 45. Organization of the Nervous System, Basic Functions of Synapses, and Neurotransmitters. In: Hall J, ed. Guyton and Hall Textbook of Medical Physiology. 12th ed. Philadelphia, PA: Saunders; 2011.
- K, Barman S, Boitano S, Brooks H. Chapter 7. Neurotransmitters & neuromodulators. In: Barrett K, Barman S, Boitano S, Brooks H, eds. Ganong's Review of Medical Physiology. 24th ed. New York, NY: McGraw-Hill Medical Publishing Division; 2012.
- Stahl S. In: Stahl S, ed. Stahl's Essential Psychopharmacology. 4th ed. Cambridge: Cambridge University Press; 2013.
- Stephenson F, Hawkins L. Neurotransmitter receptors in the postsynaptic neuron. Encyclopedia of Life Sciences. John Wiley & Sons, Ltd.; 2001. http://www.els.net/WileyCDA/ElsArticle/refId-a0000284.html. Accessed September 12, 2017.
- Hall J. Chapter 58. Behavioral and Motivational Mechanisms of the Brain—The Limbic System and the Hypothalamus. In: Hall J, ed. Guyton and Hall Textbook of Medical Physiology. 12th ed. Philadelphia, PA: Saunders; 2011.
- Meltzer H. Chapter 29. Antipsychotic agents & lithium. In: Katzung B, Masters S, Trevor A, eds. Basic & Clinical Pharmacology. 12th ed. New York, NY: McGraw-Hill; 2012.
- Buxton I. Chapter 1. Pharmacokinetics and pharmacodynamics: the dynamics of drug absorption, distribution, action, and elimination. In: Brunton L, Parker K, eds. Goodman & Gilman’s The Pharmacological Basis of Therapeutics. 11th ed. New York: McGraw-Hill; 2006.
- Nordquist N, Oreland L. Serotonin, genetic variability, behaviour, and psychiatric disorders - a review. Ups J Med Sci. 2010;115(1):2-10.
- Glennon R, Dukat M, Westkaemper R. Serotonin receptor subtypes and ligands. In: Bloom F, Kupfer D, eds. Psychopharmacology – 4th Generation of Progress. American College of Neuropsychopharmacology Web site. https://www.acnp.org/g4/GN401000039/Ch039.html. Accessed September 12, 2017.
- Tamminga C. Partial dopamine agonists in the treatment of psychosis. J Neural Transm. 2002;109(3):411-420.
- Gründer G, Carlsson A, Wong D. Mechanism of new antipsychotic medications: occupancy is not just antagonism. Arch Gen Psychiatry. 2003;60(10):974-977.
- Blumenthal D, Garrison J. Chapter 3. Pharmacodynamics: molecular mechanisms of drug action. In: Goodman L, Brunton L, Chabner B, Knollmann B, eds. Goodman & Gilman's The Pharmacological Basis of Therapeutics. 12th ed. New York: McGraw-Hill Medical; 2011.